You’re probably familiar with the effect of solid structures like buildings and trees on sound propagation. A distant dog barking within a forest has a more muffled, distorted sound compared to one barking at the far end of a flat playing field.
But what about the role of weather? Perhaps you’ve noticed the more subdued nature of noises during foggy weather or when there’s snow on the ground. Or even the more subtle differences between cold and hot conditions.
With an understanding of weather’s effect on noise pollution, predictions from mid- to long-range forecast models can be leveraged to inform planning and reduce the level of disturbance caused by noisy construction projects, festivals, and sporting events, to name a few. With MetSwift’s Claros model, such judgements may be made many months in advance.
Except for lying snow which acts kind of like a sponge for sounds, the influence of weather comes down to differences in the air’s movement, density, and particulate content. These operate through three mechanisms: refraction, atmospheric absorption, and scattering.
Refraction
Over-distance changes in air movement or temperature (via the effect on density) cause sound waves to change their direction – to be refracted, typically leading to a curved path.
These changes tend to be largest across distances in the vertical rather than horizontal. With distance from the surface, terrain features have increasingly little impact on the air, so it tends to move faster. As illustrated below, the effect is to refract soundwaves that move with the wind downward, and ones that move against the wind upward.
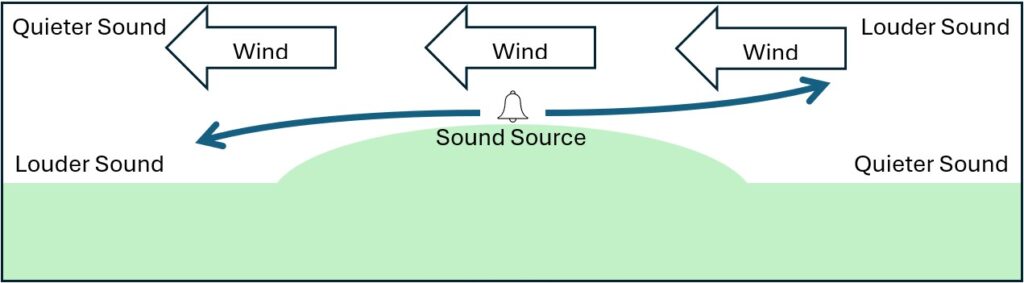
Research by DC Waddington and YW Lam of Salford University’s Acoustics Research Centre found that near-surface, noise levels can be increased by a few decibels downwind, and up to 20 decibels upwind.
Meanwhile, temperatures generally reduce with increasing height, which helps sound to move away from the surface, but under certain weather conditions, they can instead increase (temperature inversion), with the opposite effect.
Such inversions are most common when surface heating by sunshine is either absent (at night) or weak (e.g. in winter or on overcast days). In the overnight case surfaces tend to cool over time, especially when skies are clear and winds light, while the air above is slower to change temperature.
This means a noisy event taking place near dawn will tend to cause more noise pollution than one taking place in the afternoon, for example. It also tells us that the ‘dawn chorus’ of birdsong tends to seem loudest after a calm and clear night.
This effect is further enhanced if the sound travels over a body of water.
Absorption
Without getting into the technicalities, the amount of a sound wave lost to the surroundings per unit distance is mainly sensitive to the viscosity of the medium it’s travelling through. The relationship is such that sounds travel further through solids than liquids, and in liquids than gases. Some aquatic creatures use this to great effect, communicating across vast distances.
In the atmosphere, absorption mainly varies with relative humidity and temperature. An increase in either reduces the density of the air, reducing how much of a sound wave is lost to absorption, allowing sounds to travel further. However, the effect is small compared to that of refraction.
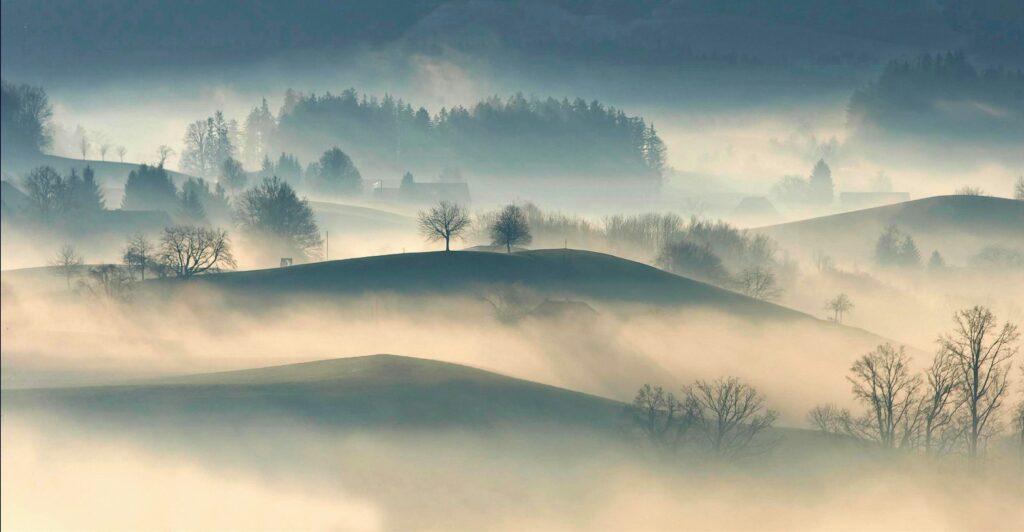
Fog occurs at 100% relative humidity; the air is fully saturated. However, because this means that water droplets exist suspended in the air, the net effect is to disperse sounds across a wider area, leading to a muffled quality and making it hard to pinpoint source(s).
Scattering
Atmospheric turbulence (movement of air in the vertical over short distances) scatters sound waves, which can diffuse noise such that it becomes more muffled where usually heard clearly, and clearer where usually muffled.
This turbulence tends to be strongest when light winds occur alongside a large heating of surfaces by sunshine.
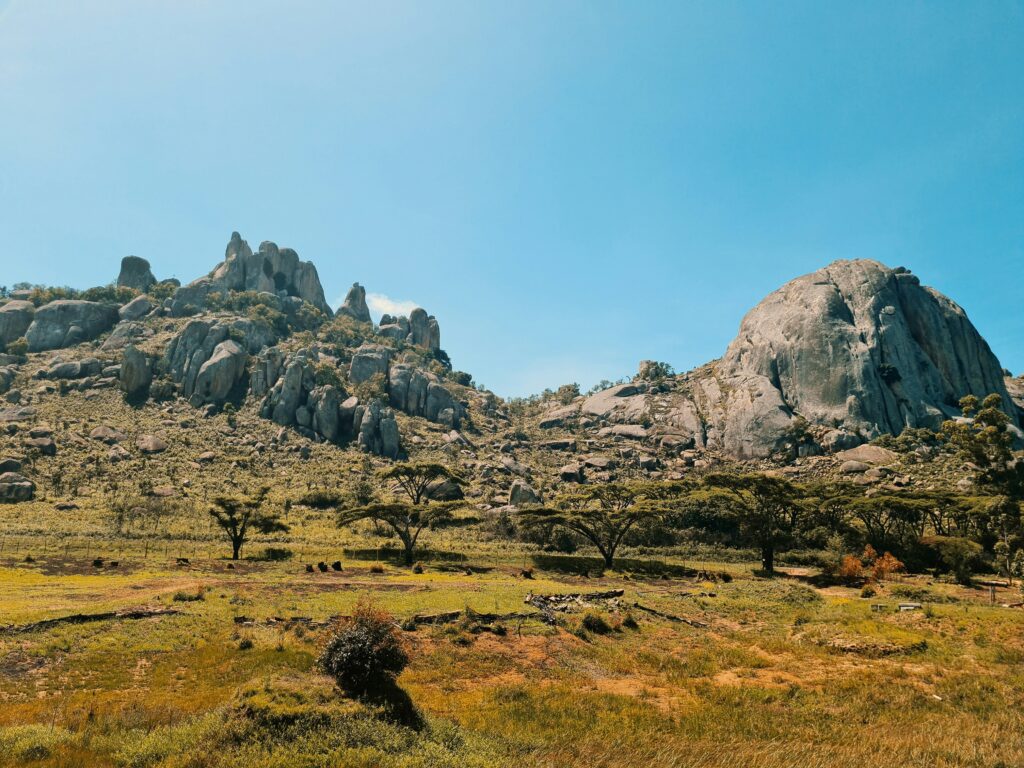
Perhaps unintuitively, particulates in the air like dust or soot usually have a negligible effect on sound waves. This becomes easier to comprehend when considering another, more tangible form of wave that occurs in large bodies of water. When people are swimming past the wave breaking zone, they bob up and down with the waves, while having no appreciable impact upon them. Similarly, particulates in the air, which must be very light to be suspended in the first place, move as the sound wave traverses them.
There may be exceptions to this in extreme circumstances, such as within a sandstorm, where particulate concentration is so high that there is more solid than gas per unit atmosphere, but I’ve not seen any confirming research on the matter.
Planning for a Less Disturbing Future
Let’s say you need to schedule a particularly noisy phase of a construction project located to the west of a quiet neighbourhood. With the knowledge of how weather affects sound propagation, it’s clear that this would best be planned for a time when westerly winds and temperature inversions are relatively infrequent.
Utilising quality assured data such as MetSwift provides, you can determine what time(s) of year are least prone to westerly winds and calm, overcast weather (when daytime inversions are most likely).
But we can’t expect every year to follow the historical averages. Using predictive models like Delos for hyper-local forecasts out to 10 days, or Claros for advanced long-range predictions, you can get a good sense how likely conditions are to deviate from average, and if so, by how much.
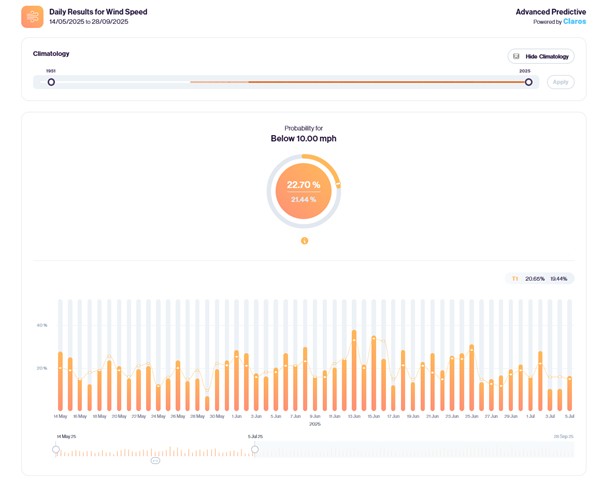
In this way, services like MetSwift offers can help you achieve onsite goals while minimising the disturbance to the wider environment, boosting your reputation in the community.
James Peacock MSc
Head Meteorologist at MetSwift